
GOLD NANOPARTICLES
Introduction
Gold (Au) nanoparticles have been investigated and technologically used since the 1800s as a result of their optical properties. Despite their decades' worth of use, it is only in recent years that researchers discovered the potential of gold nanoparticles for biological applications including those used in biological nanotechnology. A vast amount of knowledge with regards to nanotechnology has been gathered in the last decade. Despite this, researchers are still trying to understand whether the gold nanoparticles' specific properties is what makes their use extremely important. Therefore, it is essential that the intrinsic properties as well as the motivation for gold nanoparticles’ biologically related applications, especially in delivering molecules to cells, is known in order to account for such questions (Sperling et al., 2008).
The intrinsic properties of gold nanoparticles
More specifically, spherical AuNPs retain inherent properties which make it an essential bionanotechological tool, including a large surface-to-volume ratio, low toxicity, exceptional compatibility with living tissue (biocompatibility) as well as shape and size-related optoelectronic properties (electrical properties which allow the sourcing, control and detection of light)(Khlebtsov and Dykman, 2011; Sau et al., 2010). In addition to these useful attributes, AuNPs have important physical properties such as their ability to decrease the fluorescence intensity of specific substances and surface plasmon resonance (SPR) (Yeh et al., 2012).
Moreover, as the size of the core increases to 100 nm, a range of colours is exhibited by the spherical AuNPs in aqueous solutions. Generally, an absorption band can be detected between 500-550 nm. This so-called “surface plasmon band’ is absent in small AuNPs and influenced by numerous features including shape, core charge and size. As a result of the overlap between the AuNPs band and the excited fluorophore’s emission spectrum excellent decrease in fluorescence is observed (Yeh et al., 2012).
Gold Nanoparticle Synthesis
In 1951, Turkevich et al developed a synthetic method for manufacturing colloidal AuNPs 15 nm to 50 nm in diameter. To this day the Turkevich method remains one of the most widely used techniques for synthesizing AuNPs. This technique of AuNP synthesis involves the reaction of Gold (III) chloride hydrate (HAuCl4) with Trisodium citrate dehydrate (NaCt) under high temperature conditions. Throughout the years many groups have modified and improved the technique to create AuNPs with specific modifications that allow for use in many areas of interest.
The synthesis of AuNPs is dependent on nucleation, the process whereby nuclei (seeds) act as templates for crystal growth. The initial step of AuNP synthesis involves the rapid conversion of the ionic gold precursor into metallic gold nuclei within the crystal-free solution i.e. primary nucleation. These nuclei then facilitate the coalescence of small gold entities in a thermodynamically feasible manner, giving rise to the growth of AuNPs.
Gold nanoparticles (AuNPs) exhibit unique size-dependent physiochemical properties. Therefore, the protocol must be tightly regulated to ensure that the nanoparticles obtained are of exceedingly narrow size distribution, as to yield accurate and reliable performance in application. The original Turkevich method lacked the ability to yield AuNPs of consistent size. In 1973, Frens and his coworkers refined Turkevich’s method by altering the gold-to-citrate ratio to accurately control AuNP size. Frens’ method is widely used to prepare dilute solutions of moderately stable spherical AuNPs with diameters of 10 to 20 nm. This method can also be adjusted to accommodate the synthesis of larger AuNPs up to 100nm in diameter.
In order to ensure colloidal stability (a form of dispersion achieved when particles are not subject to a change in size) a charged stabilizing agent or surfactant (substance such as a detergent that reduces the surface tension, when added to a liquid) is chemically bound or absorbed at the AuNP’s surface. Upon synthesis, the gold particles which arise may either take a spherical shape, a rod-like shape or the shape of a hollow shell among others (Sonnichsen and Alivisatos, 2005; Perez-Juste et al., 2005).
In 2014, Gurunathan et al established a biocompatible protocol for synthesizing AuNPs. This green method makes use of pharmaceutically important Ganoderma, a genus of more than 300 species of wood-decaying fungi. Water-soluble AuNPs are synthesized by treating HAuCl4 with a hot aqueous extract of the Ganoderma mycelia, the mass of branched, tubular filaments (hyphae) of the fungi. This protocol is simple, cost-effective, non-toxic, and easy to use for controlling the size, shape, and stability of AuNPs. These traits are much more challenging to control when chemically synthesized nanoparticles.
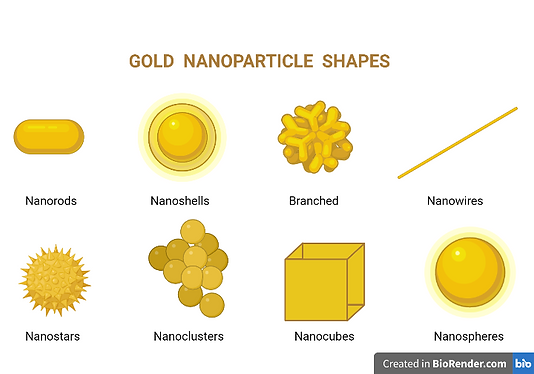
Figure 1
Here we see the different possible shapes of gold nanoparticles namely; Nanorods, Nanoshells, Branched, Nanowires, Nanostars, Nanoclusters, Nanocubes & Nanospheres.
Colloidal Gold Nanoparticles in Cancer
In studies pertaining to cancer, colloidal gold nanoparticles, which are solutions of gold nanoparticles within a fluid such as water (Voliani, 2020), have been observed to have several potential applications (Fan et al, 2020; Wang & Lu, 2017; Jain et al, 2012), especially in the areas of cancer diagnosis and imaging (Fan et al, 2020; Wang & Lu, 2017) as well as cancer therapy (Jain et al, 2012).
In cancer diagnosis and imaging, these nanoparticles can be used due to the optical characteristic they possess called surface plasmon resonance (Fan et al, 2020). This causes the nanoparticles to be able to strongly scatter and absorb light (Wang & Lu, 2017), which allows gold nanoparticles to be applied in various imaging methods such as X-ray scatter imaging, magnetic resonance imaging and fluorescence imaging (Fan et al, 2020). In fact, one method by which cancer cells can be diagnosed and visualized is through the conjugation of colloidal gold nanoparticles with monoclonal antibodies which bind specifically to receptors that are overexpressed on cancer cells (Wang & Lu, 2017). Huang et al, 2006 used this method to diagnose the presence of head and neck cancers in living tissues.
On the other hand, in cancer therapy, colloidal gold nanoparticles are used in the carrying and delivering of chemotherapeutic drugs, therapeutic genes and photosensitizers, in conducting thermal therapy and acting as radiosensitizers in radiotherapy (Fan et al, 2020; Jain et al, 2012; Wang & Lu, 2017). This method was used used to deliver the drugs cetuximab and gemcitabine to pancreatic cancer cells (Jain et al, 2012), to deliver siRNA to glioma cells to inhibit the formation of the protein Bcl2Like12 in cancer formation (Wang & Lu, 2017) and to deliver the photosensitizer Pc 4 to prostate cancer cells (Fan et al, 2020).

Figure 2
Figure of Gold nanoparticle and all of the molecules it could be complexed with
Finally, in radiotherapy, gold nanoparticles are used as radiosensitizers to enhance the effects experienced by cells (Fan et al, 2020). Since gamma and X-rays used in radiotherapy are not specific, both healthy and cancerous cells will be damaged by the radiation. However, with the introduction and concentration of nanoparticles into specific regions of the tissue, less radiation will be needed as the effects will be experienced in higher magnitude in the particle containing regions (Fan et al, 2020; Wang & Lu, 2017). Therefore, gold particles complexed with ligands that bind to overexpressed receptors on cancer cells (Wang & Lu, 2017), are injected into the tumour mass and the tumour is irradiated with X- or gamma rays causing the nanoparticles to release electrons which enhance the DNA break down within the cancer cells (Fan et al, 2020). This leads to the cells being damaged and killed (Fan et al, 2020; Wang & Lu, 2017). This method has been used in research against EMT-6 mammary carcinomas (Fan et al, 2020), MDA-MB-361 mammary adenocarcinoma and ovarian cancer (Wang & Lu, 2017).
Mechanism of Gold nanoparticles in drug therapy:
Tumour blood vessels and lymphatic vessels are distinct from normal vessels due to them being formed through the processes of angiogenesis and lymph-angiogenesis. These lymphatics and endothelial cells therefore have their own molecular markers/receptors which are different from normal and can therefore be targeted for specific drug delivery.
A specific example of said molecular markers is that of αVβ3 integrin, which tends to be highly overexpressed by various cancer cell lines. Due to integrin playing a crucial role in many vital mechanisms for cancer survival such as cell growth, migration and survival, the targeting of this molecular marker will allow the visualisation of these mechanisms as well as their specific targeting.(Kumar et al., 2013)
Mechanism for Nanoparticle communication with biological components:
A specific example of said molecular markers is that of αVβ3 integrin, which tends to be highly overexpressed by various cancer cell lines. Due to integrin playing a crucial role in many vital mechanisms for cancer survival such as cell growth, migration and survival, the targeting of this molecular marker will allow the visualisation of these mechanisms as well as their specific targeting.(Kumar et al., 2013)
Signalling molecules are utilised to target the tumours according to cell specific receptors, which further broadcast the tumours location to the NPs allowing them to come in close proximity. The nanoparticles release heat through their electromagnetic energy which in turn disrupts the tumour vessels. The nanoparticles also contain tumour specific target proteins such as tumour-targeted tissue factor (tTFs) which allow them to scavenge the tumour vessels for the presence of angiogenic tumour receptors, which in turn activate extrinsic coagulation pathways. The communication link between the nanoparticle and the coagulation pathway can be activated through the coating of the nanoparticle with fibrin recognising peptides therefore activating the pathway. Therefore different peptide coatings can be utilised to target different pathways for the treatment and diagnosis of different diseases. (von Maltzahn et al., 2011)
The nanoparticles are coated in antibodies which are specific to the cell surface receptor of the targeted tumour. The NPs can have a fully coated or partially surface which allows internalisation into the tumour cell. Example- Epidermal growth factor receptor targeted with Cetuximab. These nanoparticles get clustered in glycosphingolipid domains at the plasma membrane and are internalised via Dynamin-2 dependent endocytosis. The nanoparticle is then further internalised via actin dependent phagocytosis/pinocytosis. (Bhattacharyya et al., 2012)
Figure 3
This Figure shows the endocytosis of the Gold Nanoparticle engineered with cancer cell recognition molecules allowing the targetting of specific cells.
Colloidial Gold Nanopartices in Neurodegeneration
The major limitation often hampering effective treatment of neurodegenerative diseases (wherein progressive neuronal death occurs) is the delivery of drugs across the blood-brain barrier – a structure which separates brain tissue from the circulating blood. Clinically, the nature of the blood-brain barrier is considered a major obstacle because it renders the brain very selective in its control of drug passage, consequently restricting the potential curative effects of many promising drugs. However, Gold nanoparticles present great potential in overcoming this hurdle. In fact, they have been used not only to treat neurodegenerative diseases by drug delivery, but also to facilitate the diagnosis and monitoring of Alzheimer’s disease (Sivanesan & Rajeshkumar, 2019). Alzheimer’s disease is a neurodegenerative disease wherein aggregates of a particular protein - termed amyloid-β – are adversely deposited around brain cells. Since Gold nanoparticles have been found to have an anti-amyloid aggregating effect, they are considered to be a very promising novel treatment strategy for this disease (Shaikh et al., 2020). With regards to monitoring of Alzheimer’s, gold nanoparticles have been found to serve as great MRI contrast agents, because of the high stability and strong magnetism which they are endowed with (Vinhas et al., 2015). Diagnosis of the disease is also facilitated considering Gold nanoparticles can detect amyloid-β aggregates and semi-quantify them, which is incredibly useful in clinical cases where there is suspicion of fibrillogenesis or early protein aggregation.
Presently, clinical therapy for Alzheimer’s disease is limited to the relief of symptoms which generally accompany it. However, several recent studies have shown that even at low stoichiometric ratios, Gold nanoparticles sufficiently suppress amyloid-β aggregation. The disruption of mature amyloid-β aggregates was also possible in the presence of near-infrared laser light. Following therapy, the affected were found to exhibit an improved retention of memory and spatial learning, accompanied by a favourable increase in the level of expression of biological markers which signify neuronal survival (Sanati et al., 2019).
In addition to lowering the toxicity of amyloid-β aggregates, Gold nanoparticles were found to ameliorate oxidative stress (which is the undesirable excess of free radicals in the body), neuroinflammation, and impaired mitochondrial ATP production which are often associated with Alzheimer’s, Parkinson’s disease and Multiple Sclerosis (Lin et al., 2016) (Aghaie et al., 2019). In fact, several studies have shown that in Parkinson’s disease (a neurological movement disorder), gold nanoparticles markedly improved motor symptoms, by reducing oxidative stress and neuroinflammation. Behavioural symptoms associated with Parkinson’s disease were reverted, demonstrating the significant neuroprotective effect provided by Gold nanoparticles (Silveira et al., 2021).
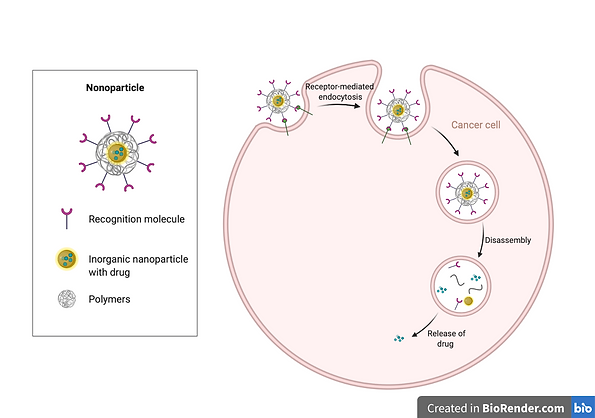
Finally, it is also important to consider that several drugs presently used to alleviate neurodegenerative disease symptoms have a low half-life, and their effects are markedly limited by low bioavailability. Therefore, by associating these medications with Gold nanoparticles, drug delivery may be improved. Consequently, metabolism of these drugs (such as L-dopa, to treat Parkinson’s, and acetylcholinesterase, to treat Alzheimer’s), can be facilitated. Considering the multitude of uses described previously, the potential of these inorganic particles in the biomedical field is very promising, especially in pursuit of innovative treatment.
Nanotechnology mechanism for drug transport across the blood brain barrier:
As previously mentioned, the blood brain barrier (BBB) is an obstacle to overcome when delivering drugs to the brain. This can be done through two pathways being either molecular or through a polymeric carrier. The molecular pathway describes the targeting of drugs to particular neuronal cells which get activated by specific enzymes within the cell, whilst the polymeric carriers may be administered intrathecally, intravenously or through implantable devices. Examples of such polymeric carriers are nanoparticles (Kreuter et al., 1995). Particularly, gold nanoparticles have been investigated for the treatment of Alzhiemers through the targeting and destruction of beta amyloid fibrils and plaques present in this neurodegenerative disorder. The mechanism of such a treatment can be described as a sort of molecular surgery whereby the gold nanoparticles are attempted to be attached to beta amyloid fibrils. Once the attachment has occurred, the mixture is incubated for several days after which exposure to microwave radiation is applied for several hours. The magnetic field of microwaves used as part of the treatment was identified to be six times smaller than that released by mobile phones, thus being harmless to healthy cells (Kogan et al., 2006). The result of this treatment was found to be effective to the extent of degrading the amyloid fibrils as well as reducing the probability of protein re-aggregation in that area (Olmedo et al., 2008).
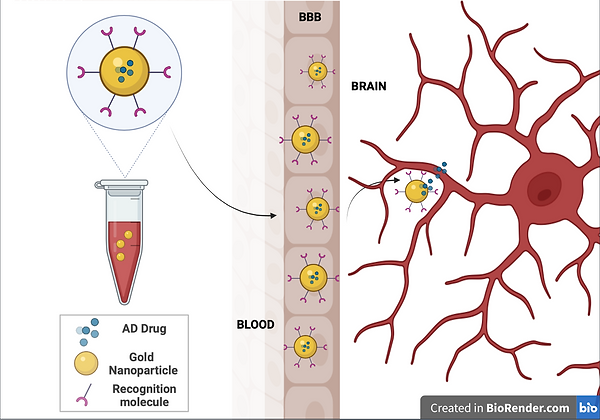
Figure 4
Here we can see the simplified mechanism of the administration of Gold nanoparticles specified to target neurodegenerative disorders due to their blood brain barrier permeability.
Drug and Gene Delivery
In recent years, nanotechnology has become one of the popular fields in science and several advances have been made. As a result, gold nanoparticles (AuNPs) have gained a lot of attention in the medical field thanks to their properties which make them highly efficient and safe at transferring molecules such as proteins, drugs or genes to their site of action (Hu et al., 2020). This section will focus mainly on the properties of AuNPs that allow them to do this, as well as on some of the practical applications of using AuNPs in drug and gene delivery.
-
Gold is inert resulting in low toxicity and good biocompatibility.
-
AuNPs can be made to any shape or size so they can be specifically produced to fit the optimal dimensions of the substance they will carry, eg. Proteins or small drug molecules.
-
AuNPs have a high surface area which makes loading easier.
-
Easily modifiable and can transfer drugs bound through physical encapsulation or through chemical bonding (Mieszawska et al., 2013).
These properties come especially useful in cancer treatments for example. The most common way of treating cancer is through oral or intravenous administration of chemotherapy drugs. However, the main issue with this is that the drugs ends up travelling throughout the whole body while only a small percentage of the drug reaches the tumour site. This can result in the treatment causing more side effects and being less effective. Therefore, researchers started looking for new drug delivery systems (DDSs) which can provide a more targeted and efficient transport of the drugs while also allowing travel of drugs through certain biological barriers, such as the blood brain barrier. Owing to the mentioned properties, gold nanoparticles (AuNPs) have proved to be ideal DDSs. When AuNPs are used as drug carriers, researchers have discovered a lowered systemic drug toxicity as well as a decreased chance of the cancer developing resistance to that specific drug (Hu et al., 2020).
Several recent studies have shown that drugs delivered by AuNPs are more effective than those administered freely. In fact, a study on hepatocellular carcinoma cells showed that drugs which were noncovalently complexed to AuNPs showed enhanced therapeutic outcomes and were also effective against chemotherapy resistant cells (Tomuleasa et al.).
Apart from transferring drugs to their site of action, AuNPs have also proven effective in the transfer of genes for the treatment or prevention of diseases. Gene therapy currently relies mainly on viral vectors which act as host cells to transfer the gene of interest. However, viruses are inflexible and typically target sites with high cytotoxicity, thus reducing the efficiency of the therapy. Conversely, AuNPs are highly modifiable while they have also proved to protect the nucleic acids by preventing nuclease degradation. An example of this being used in practice is in a study by Shahbazi et al. (2019) where they used AuNPs in combination with CRISPR to successfully held homology-directed repair in blood stem and progenitor cells (Hu et al., 2020).
AuNP Properties
Drug Delivery and Cancer
Gene Delivery

Figure 5
The application of AuNPs in Drug, Gene and Protein delivery
Conclusion
References:
-
Aghaie, T., Jazayeri, M. H., Manian, M., Khani, L., Erfani, M., Rezayi, M., Ferns, G. A., & Avan, A. (2019). Gold nanoparticle and polyethylene glycol in neural regeneration in the treatment of neurodegenerative diseases. Journal of Cellular Biochemistry, 120(3), 2749-2755. 10.1002/jcb.27415
-
Bhattacharyya, S., Singh, R. D., Pagano, R., Robertson, J. D., Bhattacharya, R., & Mukherjee, P. (2012). Switching the targeting pathways of a therapeutic antibody by nanodesign. Angewandte Chemie (International Ed. in English), 51(7), 1563-1567. 10.1002/anie.201105432
-
Brust, M., Walker, M., Bethell, D., Schiffrin, D. J., & Whyman, R. (1994). Synthesis of thiol-derivatised gold nanoparticles in a two-phase liquid–liquid system. Journal of the Chemical Society, Chemical Communications, (7), 801-802.
-
Fan, M., Han, Y., Gao, S., Yan, H., Cao, L., Li, Z., Liang, X. J., & Zhang, J. (2020). Ultrasmall gold nanoparticles in cancer diagnosis and therapy. Theranostics, 10(11), 4944–4957. https://doi.org/10.7150/thno.42471
-
Huang, X., El-Sayed, I. H., Qian, W., & El-Sayed, M. A. (2006). Cancer cell imaging and photothermal therapy in the near-infrared region by using gold nanorods. Journal of the American Chemical Society, 128(6), 2115–2120. https://doi.org/10.1021/ja057254a
-
Jain, S., Hirst, D. G., & O'Sullivan, J. M. (2012). Gold nanoparticles as novel agents for cancer therapy. The British journal of radiology, 85(1010), 101–113. https://doi.org/10.1259/bjr/59448833
-
Jana, N. R., & Peng, X. (2003). Single-phase and gram-scale routes toward nearly monodisperse Au and other noble metal nanocrystals. Journal of the American Chemical Society, 125(47), 14280-14281.
-
Jokerst, J. V., Cole, A. J., Van de Sompel, D., & Gambhir, S. S. (2012). Gold nanorods for ovarian cancer detection with photoacoustic imaging and resection guidance via Raman imaging in living mice. ACS nano, 6(11), 10366–10377. https://doi.org/10.1021/nn304347g
-
Khlebtsov, N., & Dykman, L. (2011). Biodistribution and toxicity of engineered gold nanoparticles: a review of in vitro and in vivo studies. Chemical Society Reviews, 40(3), 1647-1671.
-
Kogan, M. J., Bastus, N. G., Amigo, R., Grillo-Bosch, D., Araya, E., Turiel, A., Labarta, A., Giralt, E., & Puntes, V. F. (2006). Nanoparticle-mediated local and remote manipulation of protein aggregation. Nano Letters, 6(1), 110-115. 10.1021/nl0516862
-
Kreuter, J., Alyautdin, R. N., Kharkevich, D. A., & Ivanov, A. A. (1995). Passage of peptides through the blood-brain barrier with colloidal polymer particles (nanoparticles). Brain Research, 674(1), 171-174. 10.1016/0006-8993(95)00023-j
-
Kumar, A., Zhang, X., & Liang, X. (2013). Gold nanoparticles: Emerging paradigm for targeted drug delivery system. Biotechnology Advances, 31(5), 593-606. 10.1016/j.biotechadv.2012.10.002
-
Lin, D., He, R., Li, S., Xu, Y., Wang, J., Wei, G., Ji, M., & Yang, X. (2016). Highly efficient destruction of amyloid-β fibrils by femtosecond laser-induced nanoexplosion of gold nanorods. ACS Chemical Neuroscience, 7(12), 1728-1736.
-
Olmedo, I., Araya, E., Sanz, F., Medina, E., Arbiol, J., Toledo, P., Alvarez-Lueje, A., Giralt, E., & Kogan, M. J. (2008). How changes in the sequence of the peptide CLPFFD-NH2 can modify the conjugation and stability of gold nanoparticles and their affinity for beta-amyloid fibrils. Bioconjugate Chemistry, 19(6), 1154-1163. 10.1021/bc800016y
-
Pérez-Juste, J., Pastoriza-Santos, I., Liz-Marzán, L. M., & Mulvaney, P. (2005). Gold nanorods: synthesis, characterization and applications. Coordination chemistry reviews, 249(17-18), 1870-1901.
-
Sanati, M., Khodagholi, F., Aminyavari, S., Ghasemi, F., Gholami, M., Kebriaeezadeh, A., Sabzevari, O., Hajipour, M. J., Imani, M., Mahmoudi, M., & Sharifzadeh, M. (2019). Impact of Gold Nanoparticles on Amyloid β-Induced Alzheimer’s Disease in a Rat Animal Model: Involvement of STIM Proteins. ACS Chemical Neuroscience, 10(5), 2299-2309. 10.1021/acschemneuro.8b00622
-
Sau, T. K., Rogach, A. L., Jäckel, F., Klar, T. A., & Feldmann, J. (2010). Properties and applications of colloidal nonspherical noble metal nanoparticles. Advanced Materials, 22(16), 1805-1825.
-
Shaikh, S., Nazam, N., Danish Rizvi, S. M., Hussain, T., Farhana, A., & Choi, I. (2020). Anti-amyloid aggregating gold nanoparticles: Can they really be translated from bench to bedside for Alzheimer's disease treatment? Current Protein and Peptide Science, 21(12), 1184-1192.
-
Silveira, G. d. B., Muller, A. P., Machado-de-Ávila, R. A., & Silveira, P. C. L. (2021). Advance in the use of gold nanoparticles in the treatment of neurodegenerative diseases: new perspectives. Neural Regeneration Research, 16(12), 2425-2426. 10.4103/1673-5374.313040
-
Sivanesan, S., & Rajeshkumar, S. (2019). Gold nanoparticles in diagnosis and treatment of alzheimer’s disease. Nanobiotechnology in Neurodegenerative Diseases (pp. 289-306). Springer.
-
Sönnichsen, C., & Alivisatos, A. P. (2005). Gold nanorods as novel non bleaching plasmon-based orientation sensors for polarized single-particle microscopy. Nano letters, 5(2), 301-304.
-
Sperling, R. A., Gil, P. R., Zhang, F., Zanella, M., & Parak, W. J. (2008). Biological applications of gold nanoparticles. Chemical Society Reviews, 37(9), 1745–2140. 10.1039/b712170a
-
Vinhas, R., Cordeiro, M., Carlos, F. F., Mendo, S., Fernandes, A. R., Figueiredo, S., & Baptista, P. V. (2015). Gold nanoparticle-based theranostics: disease diagnostics and treatment using a single nanomaterial. Nanobiosensors in Disease Diagnosis, 4, 11-23. 10.2147/NDD.S60285
-
Voliani, V. (2020). Gold Nanoparticles: An Introduction to Synthesis, Properties and Applications. Berlin, Boston: De Gruyter. https://doi.org/10.1515/9781501511455
-
von Maltzahn, G., Park, J., Lin, K. Y., Singh, N., Schwöppe, C., Mesters, R., Berdel, W. E., Ruoslahti, E., Sailor, M. J., & Bhatia, S. N. (2011). Nanoparticles that communicate in vivo to amplify tumour targeting. Nature Materials, 10(7), 545-552. 10.1038/nmat3049
-
Wang, S., & Lu, G. (2017). Applications of Gold Nanoparticles in Cancer Imaging and Treatment. In M. Seehra, & A. Bristow (Eds.), Noble and Precious Metals: Properties, Nanoscale Effects and Applications. IntechOpen.
-
Yeh, Y. C., Creran, B., & Rotello, V. M. (2012). Gold nanoparticles: preparation, properties, and applications in bionanotechnology. Nanoscale, 4(6), 1871-1880.
-
Zhu, X., & Gao, T. (2019). Spectrometry. In Nano-Inspired Biosensors for Protein Assay with Clinical Applications (pp. 237-264). Elsevier.
While AuNPs have been used in the lab for hundreds of years, the true potential of these compounds has only been revealed in recent years. With applications in cancer and neurodegenerative disease treatments as well as in gene and protein delivery, it is clear that AuNPs occupy a crucial yet underacknowledged role in medicine. Furthermore, the research into these compounds is nowhere near over and it is likely that even more uses and applications for AuNPs will be unveiled.