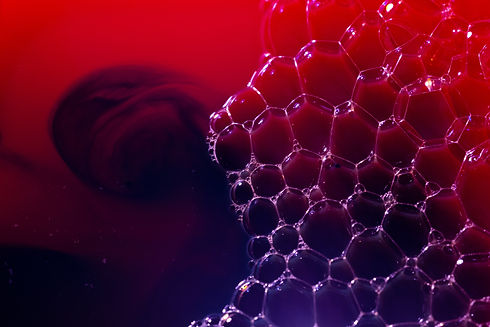
Reactive Oxygen Species
(ROS')
Introduction
Deoxyribose nucleic acid, known as DNA, is the genetic material present in all organisms that provides the information necessary to form these organisms in a complete and functional manner (Brown, 2012). These molecules have a polymeric structure which is composed of nucleotide monomers and these monomers are in turn composed of a pentose sugar, three phosphate groups and a nitrogenous base (Brown, 2012). To form the DNA polymer, nucleotides bind to each other through phosphodiester bonds to form a single strand, and in nature, DNA is observed as a double helix as two strands of nucleotide monomers wind around each other (Brown, 2012). The double helix is formed due to the formation of hydrogen bonds between nitrogenous bases present on opposite strands (Brown, 2012). In fact, there are four different nitrogenous bases which can be present within nucleotides which are adenine, guanine, cytosine as well as thymine and within the double helix, adenine always forms hydrogen bonds with thymine whilst guanine always forms hydrogen bonds with cytosine (Brown, 2012). This specificity of nitrogenous base binding is referred to as base pairing and it causes the two DNA strands that bind to each other to be complementary since the sequence of nucleotides present in one strand will determine the sequence of nucleotides of the other strand (Brown, 2012). Hence, this is how DNA is found within the cells of organisms to be able to conduct its highly essential functions. As mentioned previously, DNA provides the information necessary to form functional organisms and this is because nucleotide sequences present within its strands form the genetic code (Brown, 2012), which is used by the cells of the organism to form products required for normal cellular function (Hoy, 2013). Therefore, it is imperative that the nucleotide sequences within DNA molecules are not damaged so that cells can continue to function normally (Rowe et al, 2008).
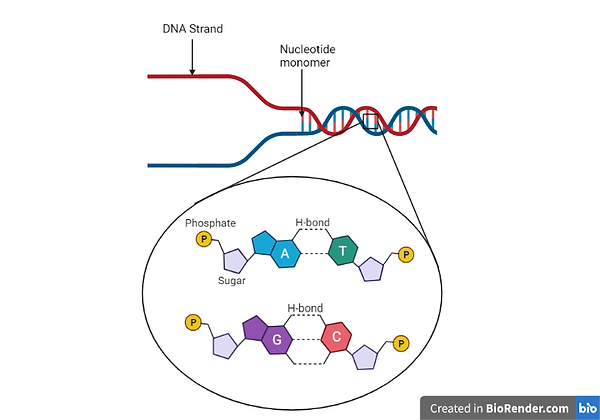
Figure 1: Image showing the structure of a DNA molecule including the nucleotide monomers making up each DNA strand and the two DNA strands which make up the DNA molecule. Moreover, the structure of nucleotides as well as the four nitrogenous bases that form the DNA can be observed along with the hydrogen bonds that are formed between specific nitrogenous bases. Created with BioRender.com.
However, multiple agents are present both within organisms as well as in the environment around the organism which cause damage to their DNA (Srinivas et al, 2019). An example of such agents are the free-radical (Srinivas et al, 2019), and non-free radical molecules (Sies & Jones, 2020), known as reactive oxygen species (ROS) (Srinivas et al, 2019). These are compounds which arise from an oxygen molecule and have an oxygen atom present within their chemical structure which is the reactive atom of the compound (Sies & Jones, 2020). Examples of ROS include hydrogen peroxide as well as the superoxide anion radical and these species can be formed either by reduction-oxidation reactions (Sies & Jones, 2020), in which a ROS molecule forms when a reducing agent donates an electron to an oxidizing agent (Nelson & Cox, 2014), or by electronic excitation (Sies & Jones, 2020), in which electrons present in the atoms of compounds move from the ground state to a high energy state to form a highly reactive atom (Bochet, 2014). ROS are produced within cells for very brief periods of time (Srinivas et al, 2019), and at these normal physiological levels, ROS have beneficial effects (Sies & Jones, 2020), such as being involved in signaling pathways within cells as well as in aerobic respiration, specifically during the electron transport chain (Srinivas et al, 2019). However, when the levels of ROS within cells rise in an abnormal manner, this will result in the cells experiencing molecular insults (Sies & Jones, 2020), one of which is damage to their DNA (Rowe et al, 2008).
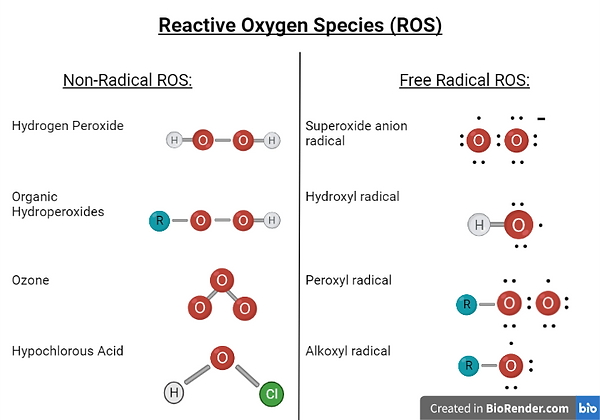.png)
Figure 2: Image showing the different examples of Non-Radical and Free Radical ROS. Created with BioRender.com.
Cellular production of reactive oxygen species (ROS)
Reactive oxygen species (ROS) also known as radicals are involved in many biochemical activities within cells and are generated by enzymatic and non-enzymatic sources. Examples of cellular activity requiring such radicals are those of signal transduction and gene transcription. Some important molecular processes involved in the production of these radicals are mitochondrial respiratory chain and lipoxygenases amongst many others such as; uncoupled nitric oxide synthase (NOS), nicotinamide adenine dinucleotide phosphate (NADPH) oxidases; xanthine oxidase; lipoxygenase & myeloperoxidase (MPO).
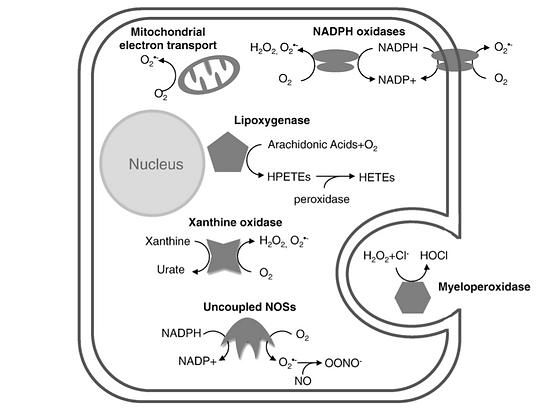
Figure 3: Image showing a diagrammatic scheme of multiple sources of ROS production within the typical mammalian cell adapted from Sugamura, K., & Keaney, J. F. (2011)
The mitochondrial respiratory chain produces a lot of electrons in its process, which are typically utilised to convert oxygen to water. But the escaping of some of these electrons may lead to the formation of oxygen radicals within the mitochondrial matrix. These oxygen radicals are typically converted into hydrogen peroxide via the enzyme manganese superoxide dismutase. The importance of this enzyme can be understood through investigation of it’s deletion, which results in prenatal fatality due to heart dysfunction.
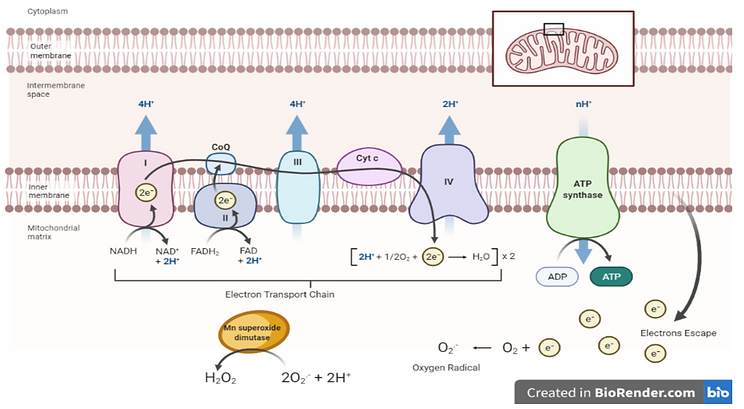
Figure 4: Image showing the mitochondrial respiratory chain and the escape of electrons into the mitochondrial matrix resulting in the production of ROS’. Created with BioRender.com.
Lipoxygenases are another subtype of enzymes which produce reactive oxygen species which are iron-containing enzymes responsible for the incorporation of oxygen within polyunsaturated fatty acids. A certain isoform of this enzyme is known to be responsible for the formation of atherosclerosis through the generation of ROS and oxidised lipids, such as oxidised-LDLs. Pathphysiologically, lipoxygenases are crucial to the inflammatory response through their role in the biosynthesis of leukotrienes, which in turn play an important role in inflammatory diseases.
ROS as a DNA Damaging Molecule
DNA damage involves DNA structural alterations which are capable of causing injury to the cells (Kaufmann and Paules, 1996). In fact, DNA damage is able to modify various mechanisms including transcription and replication, and may also result in cell death (Alexander, 1995). Among the various DNA damage mediators, ROS are well recognised mediators of deleterious effects to the DNA (Hall & PHIL, 1973).
For instance, similar to Ionisation Radiation (IR), particular ROS, most notably the water derived •OH, generated multiple DNA double-strand breaks (DSBs) as a result of damage posed to the DNA backbone (Hall & PHIL, 1973). DSBs are DNA lesion caused by endogenous or exogenous factors. Apart from DSBs, ROS have also been noted to mediate various nucleoside base oxidation, such as the formation of 8-oxo guanine (8-oxoG), which if left unrepaired directly results in DNA damage as a result of G-A or G-T transversions, mutations that alter bases from pyrimidines to purines or vice versa (Salehi, 2018; Cannan, 2014, Guo et al., 2017). Generally, oxidised bases are identified and repaired by a specific cellular DNA damage repair mechanism known as the base excision repair (BER) pathway. However, attempted BER may lead to the formation of DSBs if multiple oxidative bases occur together on opposite DNA strands (Cannan, 2014). Additionally, mitochondrial strand breaks, DNA lesions and mitochondrial DNA (mtDNA) degradation may also be induced as a result of accumulated ROS (Shokolenko et al., 2009).

Figure 5: Examples of ROS-mediated DNA damage. Created with BioRender.com.
Aside from ROS-mediated genotoxin activated damage, ROS have significant roles in oncogenic replication stress-mediated DNA damage (Srinivas, 2019).
Cellular Response to ROS
Transient fluctuations in ROS serve physiologically important functions; however, when ROS levels become elevated and/or sustained, it can elicit severe damage to cellular macromolecules such as proteins, DNA and lipids. Through evolution, cells have acquired a number of defence systems capable of combating ROS accumulation. These countermeasures include non-enzymatic molecules (e.g., glutathione, vitamins A, C, and E) as well as enzymatic scavengers of ROS (e.g., superoxide dismutases (SOD), catalase, and glutathione peroxide).
These antioxidant defence systems may become overwhelmed by sustained ROS production and fail to counteract these damaging species, triggering a state of oxidative stress. This phenomenon is thus caused by an imbalance of excess ROS over the capacity of the cell to establish an effective antioxidant response. Oxidative stress has been implicated in a number of pathologies including atherosclerosis, diabetes, pulmonary fibrosis, neurodegenerative disorders and arthritis. It is also believed that oxidative stress plays an important role in the process of ageing.
Accumulating evidence suggests that ROS serve as critical signalling molecules for a wide spectrum of cellular responses ranging from proliferation to growth arrest, to senescence, to cell death. The specific response observed reflects the balance between a variety of intracellular stress signaling pathways which are directly activated in response to ROS-mediated oxidative injury. The direct regulation of signaling molecules by ROS is termed the “oxidative interface”. Signalling pathways exhibit their phenotypic influence on the cell primarily by modulating transcription factors which in turn change the patterns of gene expression. ROS is able to interact with PI3 kinase, MAP kinases, PTEN and protein tyrosine phosphatases to alter proliferation, differentiation and survival. ROS has also been shown to interact with hioredoxin, peroxiredoxin, Ref-1, and Nrf-2 to influence ROS homeostasis and antioxidant gene regulation, and with the Ataxia-telangiectasia mutated (ATM) kinase to regulate DNA damage response. ROS is also capable of activating other signalling pathways which result in the regulation of mitochondrial oxidative stress, apoptosis, and iron homeostasis. The particular outcome of oxidative stress varies from one cell type to another. There is also variation with respect to the dosage of ROS and duration of exposure.
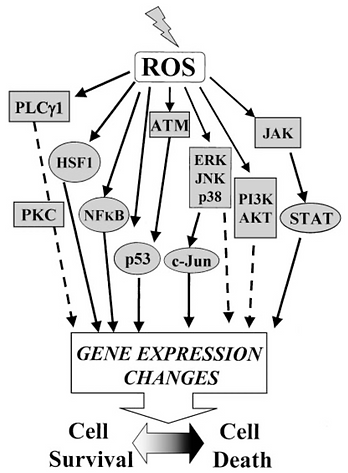
Figure 6: Image showing major signalling pathways activated by oxidative stress.
Role of ROS with DNA Damage in Cancer
Under normal conditions, Reactive Oxygen Species (ROS) are highly regulated through the expression of antioxidants. However, most cancers have shown to have abnormally high levels of ROS. Liou & Storz (2010) explain that some of the main causes for this include:
​
-
Oncogene activation – eg. K-ras gene mutation.
-
Infiltration by macrophages
-
Hypoxia and reoxygenation processes occurring in tissues.
​
These higher levels of ROS within tumour cells have been shown to increase cell growth and proliferation, promote genomic instability and cell cycle progression. They are increase cell motility resulting in cancer metastasis as well as promote angiogenesis, which is the development of new blood vessels which tumours require to grow. Furthermore, increased ROS levels are involved in the promotion of certain signalling pathways such as the MAPK/Erk 1/2 pathway and the PI3K/Akt pathway, both of which are often found to be overactivated in cancers (Liou & Storz, 2010).
​
Conversely, it is important to note that tumour cells also often have overexpression of antioxidants which are responsible for detoxifying ROS. This suggests that excessively high levels of ROS are also detrimental to tumour cells as they induce cell cycle arrest, tumour cell apoptosis and in creased cell senescence (irreversible cell cycle arrest due to telomere shortening) (Liou & Storz, 2010). In fact, chemotherapeutic agents such as platinum derivatives work by upregulating in vitro ROS levels, in the process killing both tumour cells as well as normal healthy cells (Srinivas, 2019).
ROS as Etiologies in Neurodegenerative Diseases
Reactive oxygen species (ROS) are widely viewed as chief drivers of several neurodegenerative conditions such as Alzheimer’s disease (AD), Parkinson’s disease (PD), amyloid lateral sclerosis (ALS) and Huntington’s disease (HD). Several studies have reported the involement of ROS in the progression of these conditions, as a result of the severe damaging effect they exert on neurons.
Oxidative stress in the brain triggers a cascade of events which cause downstream neuronal inflammation, tissue damage, and impaired neural proteins - which are manifested in the aforementioned neurological disorders (AD, PD, ALS and HD). While a low level of ROS is required for proper cellular function, higher levels cause significant damage to biomolecules such as lipids, DNA and proteins. Because the brain is high rich in lipid content, it is particularly vulnerable to oxidative stress. Following macromolecular damage, the loss of chemical integrity within the brain triggers programmed cell death and necrosis (Singh et al., 2019).
Considering the post-mitotic (inability to divide) state of neurons and glial cells, these neural cells are especially sensitive to ROS and have been reported to undergo apoptosis immediately following oxidative injury by excess free radicals. The biochemical composition of neural cells also makes them more prone to ROS damage, as their store of unsaturated (harbouring double bonds) lipids is highly prone to oxidative modification and peroxidation because double bonds are hot spots for free radical-attack. This oxidative damage is known to trigger a chain reaction which, in turn, causes severe damage to any neighbouring unsaturated lipids. Therefore, it is for these reasons that many scientists consider the brain to be abnormally susceptible to ROS damage. Additionally, unlike other tissues, the brain is also not particularly enriched with antioxidant defense mechanisms; it is only capable of performing about 10% of liver antioxidant activity.
The ROS heavily associated with neural damage are the superoxide anion, hydrogen peroxide, and the hydroxyl radical. In addition to biochemically modifying DNA, RNA, proteins and lipids, these species also modulate intracellular processes such as lipid peroxidation and nucleic acid oxidation. Focussing on DNA changes, hydroxyl radicals were found to be highly involved in the attack of heterocyclic bases, such as guanine. Guanine in particular, is very prone to attack by ROS, leading to the formation of one of two modified bases; 8-hydroxy-2-deoxyguanosine or 8-hydroxyguanine within the nucleus. These bases are observed at abnormally high levels in PD and AD brains. In the mitochondria, meanwhile, ROS causes oxidation of guanine into 8-oxo-deoxyguanosine. Such changes to mitochondrial DNA are directly associated with promoter inactivation and downregulation of mitochondrial gene expression, as a result of various oxidation-induced point mutations. This ultimately leads to mitochondrial dysfunction, which is causative of several neurodegenerative disorders including PD, AD and ALS(Uttara et al., 2009).
Therefore, it is clear that free radicals mediate neuropathological processes via several mechanisms; including neuroinflammation and adverse biochemical alterations to macromolecules such as DNA, protein and lipids. Therapeutic antioxidants therefore offer great potential for neuroprotection and anti-neuroinflammation in the field of neurodegenerative disease.
References
Alexander, R. W. (1995). Hypertension and the pathogenesis of atherosclerosis: oxidative stress and the mediation of arterial inflammatory response: a new perspective. Hypertension, 25(2), 155-161.
Bochet, C. G. (2014). 9.13 Organic Photochemistry. In P. Knochel (Ed.), Comprehensive Organic Synthesis (Second Edition) (pp. 330-350). Elsevier. https://doi.org/10.1016/B978-0-08-097742-3.00939-3
Brown, T. (2012). Introduction to Genetics: A Molecular Approach. Garland Science, Taylor & Francis Group, LLC.
Cannan, W. J., Tsang, B. P., Wallace, S. S., & Pederson, D. S. (2014). Nucleosomes suppress the formation of double-strand DNA breaks during attempted base excision repair of clustered oxidative damages. Journal of Biological Chemistry, 289(29), 19881-19893.
Guo, C., McDowell, I. C., Nodzenski, M., Scholtens, D. M., Allen, A. S., Lowe, W. L., & Reddy, T. E. (2017). Transversions have larger regulatory effects than transitions. BMC genomics, 18(1), 1-9.
Hall, E. J., & PHIL, D. (1973). Radiobiology for the radiologist [by] Eric J. Hall.
Hoy, M. A. (2013). DNA, Gene Structure, and DNA Replication. Insect Molecular Genetics: An Introduction to Principles and Applications (pp. 3-34). Academic Press.
https://doi.org/10.1016/j.freeradbiomed.2008.07.018
Kaufmann, W. K., & Paules, R. S. (1996). DNA damage and cell cycle checkpoints. The FASEB Journal, 10(2), 238-247.
Nelson, D. L., & Cox, M. M. (2014). Bioenergetics and
Biochemical Reaction Types. Lehninger Principles of Biochemistry (pp. 1057-1135). W.H. Freeman.
References
Rowe, L. A., Degtyareva, N., & Doetsch, P. W. (2008). DNA damage-induced reactive oxygen species (ROS) stress response in Saccharomyces cerevisiae. Free radical biology & medicine, 45(8), 1167–1177.
Salehi, F., Behboudi, H., Kavoosi, G., & Ardestani, S. K. (2018). Oxidative DNA damage induced by ROS-modulating agents with the ability to target DNA: A comparison of the biological characteristics of citrus pectin and apple pectin. Scientific reports, 8(1), 1-16.
Shokolenko, I., Venediktova, N., Bochkareva, A., Wilson, G. L., & Alexeyev, M. F. (2009). Oxidative stress induces degradation of mitochondrial DNA. Nucleic acids research, 37(8), 2539-2548.
Sies, H., & Jones, D. P. (2020). Reactive oxygen species (ROS) as pleiotropic physiological signalling agents. Nature Reviews Molecular Cell Biology, 21, 363-383. https://doi.org/10.1038/s41580-020-0230-3
Singh, A., Kukreti, R., Saso, L., & Kukreti, S. (2019). Oxidative Stress: A Key Modulator in Neurodegenerative Diseases. Molecules (Basel, Switzerland), 24(8)10.3390/molecules24081583
Srinivas, U. S., Tan, B. W. Q., Vellayappan, B. A., & Jeyasekharan, A. D. (2019). ROS and the DNA damage response in cancer. Redox Biology, 25, 101084. https://doi.org/10.1016/j.redox.2018.101084
Srinivas, U. S., Tan, B. W., Vellayappan, B. A., & Jeyasekharan, A. D. (2019). ROS and the DNA damage response in cancer. Redox biology, 25, 101084.
Sugamura, K., & Keaney, J. F. (2011). Reactive oxygen species in cardiovascular disease. Free Radical Biology and Medicine, 51(5), 978-992. 10.1016/j.freeradbiomed.2011.05.004
Uttara, B., Singh, A. V., Zamboni, P., & Mahajan, R. T. (2009). Oxidative stress and neurodegenerative diseases: a review of upstream and downstream antioxidant therapeutic options. Current Neuropharmacology, 7(1), 65-74. 10.2174/157015909787602823