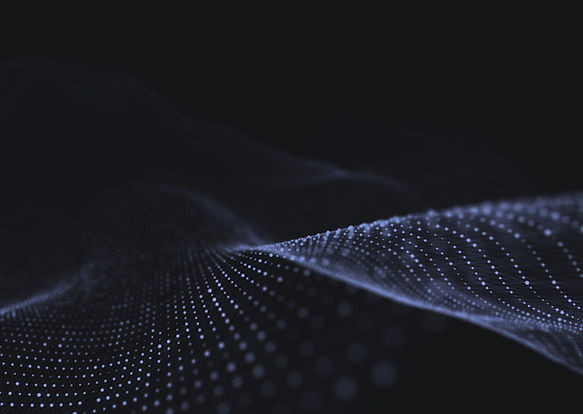
Silver Nanoparticles
What are Silver Nanoparticles?
Silver nanoparticles, also known as AgNPs, are minuscule particles of silver with sizes that typically range from 1 to 100 nM. These AgNPs can be produced in various shapes, including spherical, diamond, octagonal, and thin sheets depending on the synthesis method used (Graf et al., 2003). Their unique properties, such as size and shape, make them suitable for diverse applications, including biosensors, superconductors, antimicrobial treatments, cosmetics, and electronics (Caro et al., 2010). Furthermore, AgNPs have demonstrated promising anticancer, antidiabetic (Saratale et al., 2017), and bactericidal effects (Masimen et al., 2022). They possess a greater surface area per unit volume, which allows them to coordinate with numerous ligands. As a result, AgNPs are considered one of the most desirable nanomaterials in current biomedical research. Understanding their biological mechanisms and potentially harmful effects can contribute to their safe use in the medical field (Cassano et al., 2019; Xu et al., 2020).
The Synthesis of Silver Nanoparticles
The production of silver nanoparticles, along with other types of nanoparticles, can be classified into two main approaches (Rafique et al., 2017). The first approach, known as the "top-down" approach, involves the formation of particles from bulk materials. The second approach, referred to as the "bottom-up" approach, involves the formation of nucleation sites that develop into nanometer-sized particles. Physical synthesis methods are primarily utilised for fast and large-scale production of silver nanostructures, including ball milling, laser ablation, evaporation-condensation, arc discharge, and photoirradiation. These are known to be “bottom-up” methods (Kaabipour & Hemmati, 2021). The top-down approach has resulted in the production of silver nanoparticles with different sizes, distributions, and shapes. Chemical, physical and green reduction methods are used in the bottom-up approach for the production of silver nanoparticles. Chemical synthesis employs chemical reagents to reduce Ag cations into zero-charged Ag atoms, while green synthesis uses biological agents. Organisms and materials, such as bacteria, fungi, viruses, yeasts, plants, plant extracts, microalgae, enzymes, saccharides, and vitamins, have been used for the production of silver nanoparticles with controlled size and shape, categorised as green chemistry/synthesis (Kaabipour & Hemmati, 2021).
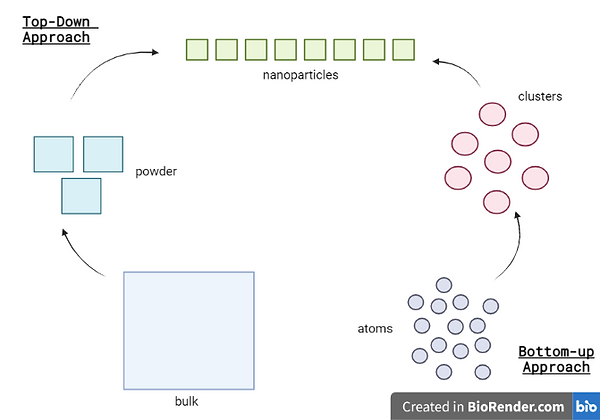
Diagram 1 - Visual representation of the top-down and bottom-up methods
While the synthesis of silver nanoparticles has been the focus of many studies, factors such as cost-effectiveness, eco-friendliness, and human well-being have often been overlooked. Chemical methods are hazardous, while physical methods are costly due to high-energy consumption. Therefore, there is an increasing interest in green synthesis techniques that use environmentally friendly materials and processes. Although alternative reagents such as ascorbic acid and sodium citrate have shown promising results, challenges such as stability, size distribution, and unknown biological functions still exist (Ahmed et al., 2016; Kaabipour & Hemmati, 2021; Natsuki, 2015).
Physical Methods
The physical synthesis of silver nanoparticles is typically carried out using mechanical and vapour-based techniques. One example of such a technique is ball milling, which involves grinding silver precursors with small steel balls in a ball mill at high speeds to create shear and impact forces that break down the precursors into smaller particles and form silver nanoparticles with controlled size, shape and composition (Xu et al., 2020). Despite its precision, ball milling has limitations such as energy consumption, the formation of agglomerated particles and its unsuitability for large-scale production.

Diagram 2 - Visualization of the ball milling process
In contrast, the evaporation-condensation method involves vaporising the metal of interest in a furnace processing chamber, which results in the production of a low-density gas phase that condenses into nuclei and grows into nanoparticles when the temperature is lowered. While this technique has the potential for large-scale production and long-term experiments, it requires expensive equipment that takes up a lot of space, has safety concerns due to high processing temperature, and consumes significant amounts of energy, which makes it economically impractical (Iravani et al., 2014; Natsuki, 2015; Rafique et al., 2017).
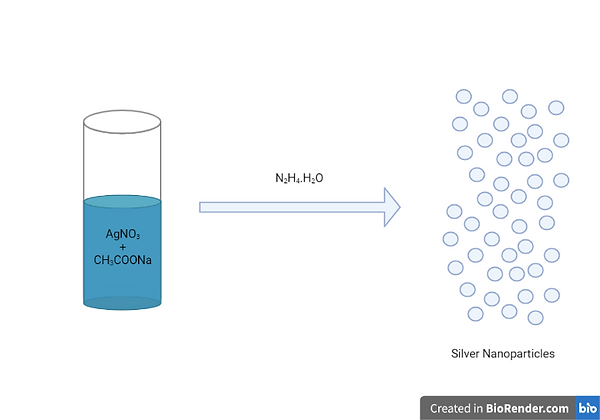
Diagram 3 - Visualisation of the evaporation-condensation method
Chemical Methods
Chemical reduction, which involves the use of a metal precursor, reducing agent, and stabiliser, is a widely used method for synthesising AgNPs. This method employs a bottom-up approach, in which the nanoparticles are formed from a nucleus of atoms rather than from the bulk. The Sol-Gel technique is a versatile chemical method for synthesising various forms of nanoparticles, including AgNPs. In this method, a gel-like mixture is prepared by mixing a silver precursor solution with a metal complex compound in a solvent, followed by heat treatment to enable nucleation and reaction to occur. However, the sol-gel method has some limitations, including the high cost of precursors, extended processing time, and difficulties with reproducibility. (Iravani et al., 2014; Rafique et al., 2017; Jadalannagari et al., 2014; Ueno et al., 2015; Thota & Crans, 2018; Milea et al., 2011).
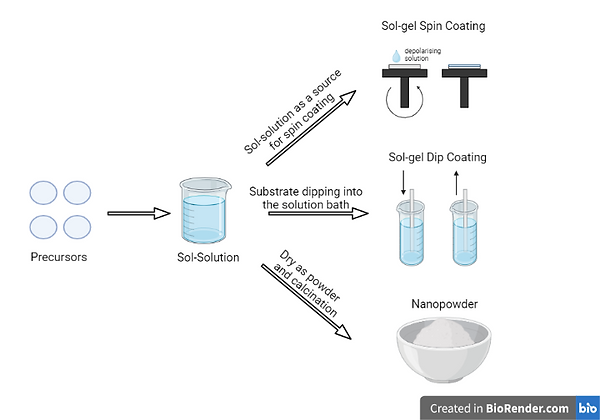
Diagram 4 - Applications of the Sol-Gel Technqiue
The Green Method – Biological Synthesis
Green synthesis of nanoparticles refers to the use of environmentally friendly methods or non-toxic reagents to produce nanoparticles. The goal of this method is to reduce environmental toxicity and health hazards. One common approach for green synthesis of AgNPs is biological synthesis, which involves reducing Ag+ to Ag0 using bio-based compounds derived from plants or organisms. This method follows a bottom-up approach similar to chemical synthesis but uses non-toxic reducing agents. Examples of biological species that have been used for this purpose include viruses, microalgae, fungi, yeast, and bacteria.

Diagram 5 - Biological Synthesis of Silver Nanoparticles
Advantages of AgNPs in Research and Medicine
In contrast to their bulk counterparts, nanomaterials have distinct physicochemical features that make them helpful in a variety of industries, including medicine. Silver nanoparticles (AgNPs) have been used to treat a variety of ailments due to research demonstrating their significant antibacterial and anticancer characteristics.
AgNPs are extremely effective at eliminating bacteria, fungi, and viruses and have a broad spectrum of antimicrobial activity. They accomplish this by a variety of processes, including bacterial cell wall breakdown, the production of reactive oxygen species (ROS), and DNA damage. AgNPs are favoured over antibiotics since bacterial resistance to them is extremely uncommon.
Breast and lung cancer among other cancers have been treated with AgNPs. By degrading the ultrastructure of cancer cells, causing the creation of ROS, damaging DNA, inactivating enzymes, or controlling signalling pathways, they can cause cancer cells to die or undergo necrosis. By halting angiogenesis, AgNPs can also stop tumour cell invasion and metastasis. AgNPs are an efficient drug delivery device because they selectively absorb into tumour cells due to the enhanced permeability and retention effect (EPR).
AgNPs can improve the immunogenicity of vaccinations and aid in bone and wound healing. They are nematicidal and anthelmintic, and they may also have anti-diabetic properties. They can be made to be small and effective, with higher doses and longer exposure intervals. Their small size permits more biological activity. AgNPs can be found in many different goods, such as scaffolds, lotions, dressings, and solvents. Although they have many beneficial effects, it is important to consider any potential toxicity issues to ensure their safe and reliable use.

Diagram 6 - Applications of Silver Nanoparticles in Research and Medicine
Toxicity and Safety Concerns of AgNPs
Numerous medical procedures use silver nanoparticles (AgNPs), such as implantable devices, drug delivery systems, and wound healing. These nanoparticles' possible toxicity is a concern, though, and further research is required to determine how safe they are. The advantages and disadvantages of AgNPs for health will be covered in this article.
The main ways that AgNPs can enter the body are by skin contact, inhalation, ingestion, and injection. These nanoparticles can be detected in trace amounts in the skin as well as in organs like the kidneys, brain, stomach, lungs, liver, and heart. AgNP deposits have the potential to be hazardous and can cause genetic mutations, necrosis, or even apoptosis. For instance, it has been discovered that AgNP lung deposits can cause asthma and pneumonia.
AgNPs can pass through barriers like the blood-testis barrier (BTB) because of their small size. As a result, male organisms have decreased fertility and their offspring exhibit teratogenicity. AgNPs' inherent qualities have nothing to do with their propensity for toxicity. More silver ions (Ag+) will be released from the nanoparticles with a higher surface area, increasing their potential toxicity. The amount of toxicity is influenced by variables like dosage, concentration, and exposure duration.
It would be beneficial that in vivo research be done to better understand the pharmacodynamic characteristics of AgNPs. The creation of safe and biocompatible agents may result from this. AgNPs have many medical uses, but they must be handled with extreme care to prevent toxicity from jeopardising the patient's health. To completely appreciate the features of AgNPs, more investigation is necessary into both their potential health advantages and hazards.It is advised that in vivo research be done to better understand the pharmacodynamic characteristics of AgNPs. The creation of safe and biocompatible agents may result from this.
Applications of Silver Nanoparticles
Antibacterial activity
The Antimicrobial activity of silver nanoparticles against microorganisms such as bacteria, viruses, and fungi are being established. These antimicrobial properties occur due to their large surface area to volume ratio, which facilitates their interaction with the microbial cell membrane and disrupts cellular processes, leading to cell death, according to Baker et al., (2005). The antibacterial effectiveness of nanoparticles against E. coli was investigated in both liquid and solid medium. At low concentrations, the nanoparticles were found to have antibacterial activity. At a concentration of 8 g/cm2, the nanoparticles were found to be cytotoxic to E. coli cells. The mechanism underlying silver nanoparticle antibacterial activity was considered to be related to nanoparticle surface area to volume ratio. The smaller sized particles possessed larger surface area to volume ratio and hence efficient antibacterial activity. Thus, the nanoparticles were found to be cytotoxic to E. coli. cSilver nanoparticles can generate ROS that further enhance their antimicrobial activity. Matsumura et al. (2003) suggested two possible processes involved in the action of silver zeolite: first the bacterial cells coming in contact with silver zeolite take in silver ions which damage the bacterial cell. Secondly, the generation of reactive oxygen species through inhibition of respiratory enzymes by silver ions damages the bacterial cell itself.
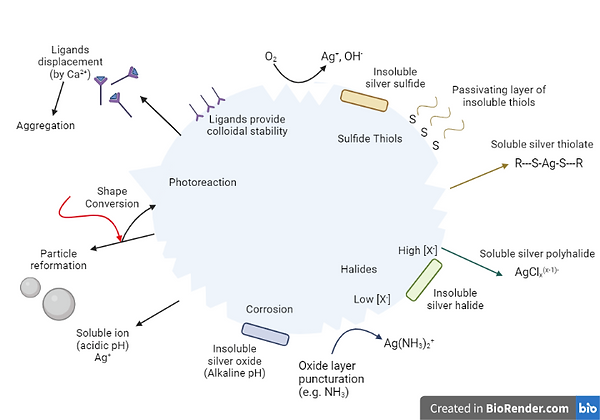
Diagram 7 - The use of silver nanoparticles in antibacterial activity
Wound Healing
The use of silver in wound management dates back thousands of years. Around Egyptian times in 1850 BC, silver was administered to wounds; also, Hippocrates' textbooks described the beneficial effects of silver in wound healing. Silver-based lotions and ointments, as well as AgNPs-based biomedical goods such as wound dressings, are now commercially available for a variety of medical applications due to their broad-spectrum antibacterial capabilities. Dai et al. (2016) conducted an experiment in which they developed an antimicrobial peptide-AgNPs composite and evaluated its wound healing properties in vivo on a diabetic rat model, demonstrating improved wound healing without side effects on dermal tissues and enhanced interactions between peptide and lipopolysaccharide moieties on bacteria, indicating a broad spectrum of activity without inducing bacterial resistance. Silver's antibacterial qualities significantly lower the likelihood of microbes developing resistance and boost the efficiency against multi-drug-resistant pathogens. Kumar et al. investigated the wound healing properties of AgNP in albino rats using wound models. The animals were given cream formulations containing varying percentages of AgNPs, and they demonstrated reduced wound area, higher collagen deposition, less macrophages, tissue oedema, and more fibroblasts at the highest concentration of silver.
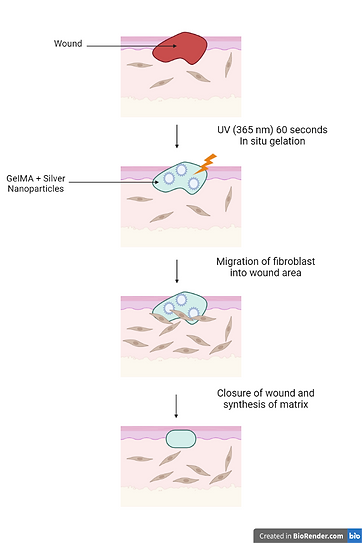
Diagram 8 - Silver nanoparticles and wound healing
Bioimaging
Because of their high enhancement factor and biocompatibility, AgNPs are frequently used as the substrate for SERS-based bioimaging. SERS is a strong analytical technique that uses metallic nanoparticles like silver nanoparticles (AgNPs) to magnify Raman signals from molecules adsorbed on their surface. SERS imaging with AgNPs has been used to identify and image cancer cells, where AgNPs conjugated with targeting ligands can bind to cancer cells preferentially and provide a molecular image of the cells. Wang et al. (2019) created a SERS-based imaging system using AgNPs functionalized with aptamers that bind to breast cancer cells precisely. The study indicated that the SERS imaging system had a high sensitivity and specificity for detecting and visualising single breast cancer cells.
Drug Delivery
On mice, AgNPs were assessed as drug carriers for breast cancer. For the first time, systemically administered AgNPs are effective in slowing the growth of solid, TNBC mammary tumours in mice, implying that AgNPs could be useful in the treatment of some human breast cancers. Notably, cytotoxic AgNPs to TNBC cells in vitro do not produce toxicity or disturb homeostasis in non-malignant breast epithelial cells. We show that the TNBC selective cytotoxic property of AgNPs is dependent on cell exposure to intact AgNPs but is unaffected by particle size, shape, or capping agent. The TNBC‐selective cytotoxicity of AgNPs is not shared by ionic silver; it can be considered a new to nano” cytotoxic property. The bulk of the studies employed AgNPs with only two components: silver and a dense stabilising layer of PVP, a polymer considered generally safe by the US Food and Drug Administration. This simplicity, when combined with existing simple and scalable production capabilities, makes them appealing for further development." Silver nanoparticles have been shown to cause cytotoxicity in a variety of cell types via apoptosis and necrosis. Furthermore, they show results against secondary effects of current therapies, such as DNA damage, the generation of reactive oxygen species (ROS), increased leakage of lactate dehydrogenase (LDH), and the inhibition of stem cell differentiation. Another important application of silver nanoparticles developed for antiviral purposes concerns influenza treatment. A considerable number of results indicating the inhibitory efficacy of these nanoparticles against the H1N1 virus, followed by the H3N2 virus, have been reported. A nanoscale delivery system for zanamivir medicine, which is used to treat and prevent influenza, was created alongside silver nanoparticles using a simple chemical procedure involving a vitamin C solution. Surface enrichment of AgNPs with amantadine was created using a similar strategy to overcome drug resistance demonstrated by the H1N1 virus. When zanamivir and amantadine were combined on the surface of AgNPs, they inhibited neuraminidase and hemagglutinin activity more effectively than single-drug treatments. Furthermore, structural changes in MDCK host cells caused by H1N1 viral invasion were significantly reduced by inducing DNA fragmentation, chromatin condensation, and caspase-3 activation using the zanamivir and amantadine nanosystems in combination with AgNPs.
Silver nanoparticles (AgNP) have an array of applications in various fields of medicine and science. In more recent years, silver nanoparticles have gained more and more attention due to their distinctive physical, chemical and biological properties. Furthermore, their relatively small size and high surface area give rise to properties such as increased reactivity and more efficient antimicrobial activity, further contributing to their importance in the medical field. Nonetheless, more studies must be conducted on silver nanoparticles as there are still concerns regarding their toxicity and their environmental effect. Thus, more rigorous guidelines must be set out for the production, use and storage of silver nanoparticles to avoid any risks that may arise from AgNPs for both the environment and human health.
References
Abbasi, E., Milani, M., Fekri Aval, S., Kouhi, M., Akbarzadeh, A., Tayefi Nasrabadi, H., Nikasa, P., Joo, S. W., Hanifehpour, Y., Nejati-Koshki, K., & Samiei, M. (2016). Silver nanoparticles: Synthesis methods, bio-applications and properties. Critical Reviews in Microbiology, 42(2), 173-180. https://10.3109/1040841X.2014.912200
Advani Suresh, G., & Hsiao Kuang-Ting. (2012). Manufacturing Techniques for Polymer Matrix Composites (PMCs). Woodhead Publishing. https://10.1533/9780857096258
Ahmed, S., Ahmad, M., Swami, B. L., & Ikram, S. (2016). A review on plants extract mediated synthesis of silver nanoparticles for antimicrobial applications: A green expertise. Journal of Advanced Research, 7(1), 17-28. https://10.1016/j.jare.2015.02.007
Caro, C. (2010). Silver Nanoparticles : Sensing and Imaging Applications. (). IntechOpen. https://10.5772/8513
Cassano, D., Mapanao, A., Summa, M., Vlamidis, Y., Giannone, G., Santi, M., Guzzolino, E., Pitto, L., Poliseno, L., Bertorelli, R., & Voliani, V. (2019). Biosafety and Biokinetics of Noble Metals: The Impact of Their Chemical Nature. ACS Applied Bio Materials, 2(10), 4464-4470. https://10.1021/acsabm.9b00630
Cui, B., Zheng, L., Waryoba, D., Marinescu, M., & Hadjipanayis, G. (2011). Anisotropic SmCo 5 flakes and nanocrystalline particles by high energy ball milling. Journal of Applied Physics, 109(7), 07A728-3. https://10.1063/1.3562447
Graf, C., Vossen, D. L. J., Imhof, A., & van Blaaderen, A. (2003). A General Method To Coat Colloidal Particles with Silica. Langmuir, 19(17), 6693-6700. https://10.1021/la0347859
Iravani, S., Korbekandi, H., Mirmohammadi, S. V., & Zolfaghari, B. (2014). Synthesis of silver nanoparticles: chemical, physical and biological methods. Research in Pharmaceutical Sciences, 9(6), 385-406. https://www.ncbi.nlm.nih.gov/pubmed/26339255
Jadalannagari, S., Deshmukh, K., Ramanan, S. R., & Kowshik, M. (2014). Antimicrobial activity of hemocompatible silver doped hydroxyapatite nanoparticles synthesized by modified sol–gel technique. Applied Nanoscience, 4(2), 133-141. https://10.1007/s13204-013-0197-x
Kaabipour, S., & Hemmati, S. (2021). A review on the green and sustainable synthesis of silver nanoparticles and one-dimensional silver nanostructures. Beilstein Journal of Nanotechnology, 12(1), 102-136. https://10.3762/bjnano.12.9
Khan, M., Shaik, M. R., Adil, S. F., Khan, S. T., Al-Warthan, A., Siddiqui, M. R. H., Tahir, M. N., & Tremel, W. (2018). Plant extracts as green reductants for the synthesis of silver nanoparticles: lessons from chemical synthesis. Dalton Transactions : An International Journal of Inorganic Chemistry, 47(35), 11988-121. https://10.1039/c8dt01152d
Lee, J., Yang, J., Kwon, S. G., & Hyeon, T. (2016). Nonclassical nucleation and growth of inorganic nanoparticles. Nature Reviews. Materials, 1(8)https://10.1038/natrevmats.2016.34
Masimen, M. A. A., Harun, N. A., Maulidiani, M., & Ismail, W. I. W. (2022). Overcoming Methicillin-Resistance Staphylococcus aureus (MRSA) Using Antimicrobial Peptides-Silver Nanoparticles. Antibiotics (Basel), 11(7), 951. https://10.3390/antibiotics11070951
Milea, C. A., Bogatu, C., & Duta, A. (2011). THE INFLUENCE OF PARAMETERS IN SILICA SOL-GEL PROCESS. Bulletin of the Transilvania University of Braşov. Series I Engineering Sciences, 4(1), 59. https://search.proquest.com/docview/1010406392
Mostafa, A. A., Sayed, S. R. M., Solkamy, E. N., Khan, M., Shaik, M. R., Al-Warthan, A., & Adil, S. F. (2015). Evaluation of Biological Activities of Chemically Synthesized Silver Nanoparticles. Journal of Nanomaterials, 2015, 1-7. https://10.1155/2015/789178
Nanda, Anima, MSc, PhD, & Saravanan, M., MSc, MPhil. (2009). Biosynthesis of silver nanoparticles from Staphylococcus aureus and its antimicrobial activity against MRSA and MRSE. Nanomedicine, 5(4), 452-456. https://10.1016/j.nano.2009.01.012
Natsuki, J. (2015). A Review of Silver Nanoparticles: Synthesis Methods, Properties and Applications. International Journal of Materials Science and Applications, 4(5), 325. https://10.11648/j.ijmsa.20150405.17
Rafique, M., Sadaf, I., Rafique, M. S., & Tahir, M. B. (2017a). A review on green synthesis of silver nanoparticles and their applications. Artificial Cells, Nanomedicine, and Biotechnology, 45(7), 1272-1291. https://10.1080/21691401.2016.1241792
Rafique, M., Sadaf, I., Rafique, M. S., & Tahir, M. B. (2017b). A review on green synthesis of silver nanoparticles and their applications. Artificial Cells, Nanomedicine, and Biotechnology, 45(7), 1272-1291. https://10.1080/21691401.2016.1241792
Saratale, G. D., Saratale, R. G., Benelli, G., Kumar, G., Pugazhendhi, A., Kim, D., & Shin, H. (2017). Anti-diabetic Potential of Silver Nanoparticles Synthesized with Argyreia nervosa Leaf Extract High Synergistic Antibacterial Activity with Standard Antibiotics Against Foodborne Bacteria. Journal of Cluster Science, 28(3), 1709-1727. https://10.1007/s10876-017-1179-z
Simchi, A., Ahmadi, R., Reihani, S. M. S., & Mahdavi, A. (2007). Kinetics and mechanisms of nanoparticle formation and growth in vapor phase condensation process. Materials in Engineering, 28(3), 850-856. https://10.1016/j.matdes.2005.10.017
Thanh, N. T. K., Maclean, N., & Mahiddine, S. (2014). Mechanisms of Nucleation and Growth of Nanoparticles in Solution. Chemical Reviews, 114(15), 7610-7630. https://10.1021/cr400544s
Thota, S., & Crans, D. C. (2018). Methods for Preparation of Metal Nanoparticles. Metal Nanoparticles (pp. 15-31). John Wiley & Sons, Incorporated. https://10.1002/9783527807093.ch2
Ueno, S., Nakashima, K., Sakamoto, Y., & Wada, S. (2015). Synthesis of Silver-Strontium Titanate Hybrid Nanoparticles by Sol-Gel-Hydrothermal Method. Nanomaterials, 5(2), 386-397. https://10.3390/nano5020386
Ullah, M., Ali, M. E., & Abd Hamid, S. B. (2015). ChemInform Abstract: Structure-Controlled Nanomaterial Synthesis Using Surfactant-Assisted Ball Milling - A Review. ChemInform, 46(7), no. https://10.1002/chin.201507288
Xu, L., Wang, Y., Huang, J., Chen, C., Wang, Z., & Xie, H. (2020). Silver nanoparticles: Synthesis, medical applications and biosafety. Theranostics, 10(20), 8996-9031. https://10.7150/thno.45413